Notes on Batteries
Portable power seems to be one of those areas of "black magic", at least at the hobbyist-level of the electronics community. Almost everybody is familiar with paradigm of double-A's and disposable cells in general, but rechargeable cells tend to be treated as "those batteries that are really expensive and you can plug in to recharge".
A while back I found myself frustrated at the apparent lack of clear and concise online resources detailing the proper use of various batteries - even Wikipedia (usually a reverable font of knowledge) was vague at best. I figured, if nobody else was going to look into this, why not me?
Sadly, I am not a professional in this area - I am not an expert and my opinions should not be taken as hard fact without experimental evidence to back them up. What I have tried to do here is compile information from all the resources I could get my hands on, and document what appear to be the 'general consensus'/best practices/industry standards. Where I can I reconcile the anecdotal (and sometimes mythical) rules of thumb that I find with experimental data or specs from manufactures.
tl;dr - I know nothing. If you kill yourself, it's your fault.
WARNING: All of the following info applies to the various cell chemistry's in general - a cells manufacturer and/or datasheet has veto power over any and all of these numbers and methods.
Resources and Credit
BB Battery - Lead-acid battery manufacturer. I see their products pop up everywhere.
Battery University - Not actually a university. Battery information site, appears to be associated with the company Cadex.
AA Power Corp/Battery Space - Webstore, but has some useful info in some product descriptions and datasheets.
The DIY UPS - Interesting case study on uninterrupted power supply design, with useful notes on lead-acid batteries.
Terminology
Chemistry - A description of the chemical mechanism contained within a cell that actually produces the power.
Cell - The base unit. A single cell will always produce the nominal voltage for its chemistry, but different cells may have different capacities.
Battery - A collection of cells. May also be called a 'pack'. Usually described with a phrase similar two 'xSyP' where x and y are integers. This indicates that there are x subpacks in series, and each subpack has y cells in parallel.
Capacity (C) - A value that gives a rough estimate of the amount of power a battery or cell can provide, given in Ampere-Hours (Ah). In theory, the cell/battery in question can expected to provide that amount of current for an hour. In practice, the capacity of a cell/battery may be affected by temperature, age, or mechanical limitations that limit rate of discharge.
Lead-Acid
The lead-acid chemistry is old, widely available, and is pretty much the lowest energy density you can buy. Lead-acid cells fall into two general categories: Valve Regulated Lead Acid (VRLA) (often called Sealed Lead Acid (SLA)) and Flooded. Flooded batteries have a liquid electrolyte (traditionally a mix of sulphuric acid and water) which occasionally needs topping up due to evaporation and electrolysis. A car battery is an excellent example of a flooded battery. VRLA batteries either have the electrolyte mixed with a thickener (in the case of the so-called 'gel cell') or absorbed in a mat of glass fibers (in the case of the Absorbed Glass Mat (AGM) cell) rendering the electrolyte (mostly) immobile. Unlike flooded batteries, VRLA batteries can usually be transported in any orientation (including upside down) and require no topping up or other maintenance. Most manufacturers discourage the use of VRLA batteries when inverted, as safety vents are placed along the top of the battery. If the battery was to vent gas in an emergency while inverted, there is a chance it may also vent electrolyte.
caution: Due to the electrolysis of the electrolyte when charging, charging lead-acid cells will produce hydrogen and oxygen gas. VRLA cells are designed to contain and recombine the hydrogen and oxygen gas, however if the battery is badly overcharged it may vent excess gas. Flooded cells will vent some gas in the course of normal charging. Needless to say, care should be taken to avoid igniting this vented gas.
Charging & Terminating Charge
VRLA and Flooded batteries have identical charge requirements - despite their mechanical differences, the same mechanisms operate both. That said, different brands and types of batteries will usually have slightly different charge requirements. Fortunately the simplicity of this chemistry makes it incredibly forgiving - the following numbers should fall within the margins of error of pretty much all commercially available lead-acid batteries:
Nominal voltage is usually listed as 2 volts per cell (12 volts in a six cell battery); in reality it's usually closer to 2.3 volts per cell (13.8 volts in a six cell battery).
- Cycle charge: Charge the battery at 2.450 volts per cell (14.7 volts for a six cell battery) at 0.1C. Disconnect when voltage across battery terminals has stabilised at 2.450V/cell. Some overcharge is fine (in fact, it may actually charge the battery further), but leaving it for long periods (say, several days) risks excessive gas build up.
- Trickle charge: Charge battery at 2.275 volts per cell (13.65 volts for a six cell battery) at 0.1C. May be left connected indefinitely. Useful for standby power supplies. May also be known as 'float charge'.
Cells should not be discharged past 2.10 volts per cell (12.6 volts in a six cell battery). Any battery that falls back to less than 2.10V/cell within 24 hours after charging can be considered dead.
Maintenance
Refer to the user manual of the cell/battery manufacturer for instructions and guidelines for topping up electrolyte (if needed).
Avoid leaving lead-acid cells in a discharged state - the electrodes will "sulfinate"/"sulfate" and the battery will eventually be useless. It may be possible to bring the batteries back by running them through several cycles of full depletion and full cycle charge.
Similarly, a trickle charge is designed to never quite fully charge the battery and thus leaving a lead-acid battery on this charge method for long periods may cause also "sulfination". Bringing the charge voltage up to the cycle charge level for a few hours every six months will probably ward this off.
(Quick and Dirty) Worked Example
I recently got my hands on a huge pile of 12V nominal, 17Ah SLA batteries - old cartridges for APC UPS's. Among them were two BB Battery BP17-12 batteries.
The manual recommends 2.275V/cell for standby charging, and 2.450V/cell for cycle use, and with the current limited to 0.1C.
The capacity in this case is 17Ah, ergo the charging current would be: 0.1 * 17 = 1.7 amps.
This battery has six cells (12V / ~2V-per-cell = 6) so the voltage for cycle charging would be: 2.450V * 6 = 14.7V. For standby charging: 2.275V * 6 cells = 13.65V.
So if I build a power supply that delivers 1.7 amps at 14.7V or 13.65V, I'll have a functional battery charger.
My solution to this was to build a circuit around an LM317T linear regulator. The LM317 is a common/inexpensive adjustable regulator and the 'T' variant delivers up to 1.5 amps. So, yes, I am fudging it a bit: I'm using the max output of the regulator as the current limiter, and I am not quite getting up to 1.7 amps. I'm doing this because the next step up in the LM317 series is LM317K which delivers 5 amps and would require a large resistor to drop the current to an acceptable level. I could wire up two LM317T's, but I would need to be very careful with the voltage adjustment of both and would still need a resistor to limit the current.
Here's a circuit diagram:
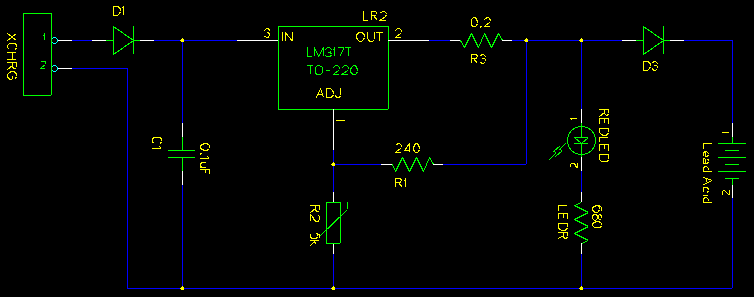
REDLED and LEDR are optional, but useful. LEDR has been calculated for 15V, so REDLED may be a bit dim.
D1 protects the circuit if the external power supply is plugged in backwards. D3 is in place to prevent the battery from discharging back through the circuit when the charger is switched off. D1 and D3 both need to be of sufficient rating to handle the voltage and currents used. I happened to have a bunch of MUR405 rectifier diodes in my junk box; they are rated up to 4 amps which is more than enough for this purpose.
R1 and R2 actually set the output voltage. Note that R2 is adjustable - I find using a variable resistor much easier than trying to nail the needed resistance exactly with discrete resistors. This also allows you to adjust for environmental conditions and odd voltage drops through D3. If you want to be fancy R2 can be a potentiometer and mounted externally - I opted for a precision adjustable resistor. (Incidentally, it turned out to be the most expensive part of the whole circuit.)
R3 is recommended by the Fairchild Semiconductor datasheet for the LM317T to set the output impedance.
C1 is just there to filter out noise from the power input. It is recommended by the Fairchild Semiconductor datasheet for the LM317T if the regulator is more than 15cm away from the primary power supply.
XCHRG is the connection to any DC power supply that provides more than 1.5 amps and up to 37V. (Input voltage must be above the output voltage.)
Note that I may have the battery symbol the wrong way 'round on the diagram; in any case, on the current diagram pin 1 is positive and pin 2 is ground.
Nickle-Cadmium (NiCad)
Nickle Cadmium cells are a bit maligned due to the toxicity of their negative electrode. In the industrial arts Cadmium has a reputation of being particularly insidious: unlike many other metals whose vapours would make a worker physically and obviously sick, Cadmium poisoning is only detectable by (presumably) a blood test or when the worker in question suddenly keels over dead at the welding booth.
That said, the Cadmium chemistry probably isn't going anywhere. Modern NiCad cells have a higher cycle life than just about anything else out there (Cadex claims to have tested a cell that had over 95% capacity after 2300 cycles - the test was discontinued due to time constraints), are only slightly more expensive than lead-acid (the de-facto standard of cost due to simplicity and age of technology), operate over a wide temperature range, prefer being repeatedly and rapidly drained and charged, and have a shelf life measured in years.
Downsides? The energy density isn't all that great (only slightly higher than Lead-Acid) and they're toxic.
The new Lithium-Iron-Phosphate chemistry is poised to take over NiCads niche (comparable cycle life, much higher energy density, less unstable than traditional Lithium-Ion chemistry, better for the environment) but production is still ramping up and manufacturers are probably going to be hesitant to replace a tried-and-true technology.
(There is a ton of information about NiCads here, including notes on various edge cases and tricks. Most of the information from this page is pulled from that link.)
Quick and Dirty Charging
Charge voltage seems to be in the 1.45 to 1.5 voltage range. This range is from information available at Battery University and the Inspecting and Charge Method sections at Wikipedia's NiCad artical. However, my tests with a multimeter and a mobile phone indicate a charge voltage of about 1.9V per cell - significantly higher. It may be that there isn't really a specific voltage to adhere to when charging - it may be that the current is much more important. I'm sure there is an upper limit to the voltage (arcing between electrodes is probably a no-no, for example) but the only side effect may be increased heat/pressure in the cell (both of which should be kept as low as possible, natch). If anybody can give me insight into this, I'd be much obliged.
One of the 'gotchyas' of NiCads is that the efficiency of charge (that is, how much of the supplied current the battery actually absorbs over a given time frame) goes down as the percent of C used to charge the battery is reduced. Generally speaking, a healthy NiCad will have a charge efficiency of about 90% at 1C (that is, a 1000mAH cell being charged at 1000mA will accept 900mA over the course of one hour, and thus would need to charged for about 1.1 hours ) and around 70% at around C/10. Notes that the relationship isn't linear. NiCad cells tend to have an efficiency of about 100% for the first 70% or so of charge. Since all the energy is absorbed there is little to no heat build up and currents several times that of the cells C-rating can be applied to the cell. Many "ultrafast" chargers exploit this to charge a cell to 70% of capacity within minutes, and then reduce the charge rate for remaining 30% of charge.
It's worth pointing out that heat is the enemy of charging NiCads. A cell at an elevated temperature will trip temperature-unrelated end-of-charge detection in many chargers before the cell is actually 'fully' charged.
So:
- "Ultrafast" charge: Dump several times the C-rating into the cell until it its 70% charged (usually indicated by heating). Charger will then switch to a different charge method to fill the cell up the rest of the way. I wouldn't recommend this method as a general charging method as the exact multiple of the C-rating that the cell can safely withstand is dependent on the cell.
- "Fast" charge: Apply 1C current to the cell. Simple and relatively quick.
- "Overnight" charge: This tends to be described as C/10 for anywhere from 12 to 16 hours.
- "Trickle" charge: Usually listed as charging at rates between 0.05C and 0.1C indefinitely.
Please note that the naming of the above charge-types is completely arbitrary and is for reference purposes only. It may or may not line up with references from other documents.
Trickle charging is somewhat counter-intuitive. The general consensus seems to be that NiCads shouldn't be left charging (including trickle charging) for long periods of time in order to prevent heating/"crystallization"/"memory effects". This seems to jive with what (little) I know about the characteristics of NiCad cells, although the definition of 'long periods of time' tends to be vague. The only time tickle charging seems to be required/recommended is when breaking in battery packs that are new or have been in storage for a long time in order to bring the charge of all the cells in the pack up to approximately the same level.
Frankly, cylinders (which tend to be assembled as cheaply as possible) containing hot heavy metals and high pressure gasses make me nervous. I would pull the cell off the power source as soon as the math indicates that it should be charged, stick with Fast Charge method only, and avoid charging cells/packs that are only partially discharged.
Charging a pack of NiCads is fairly straight forward. Assuming the xSyP notation, to determine the charge voltage, multiply the voltage per cell by x. To determine the charge current (and to calculate the capacity of the whole pack) - multiply the capacity of a single cell by y.
Terminating Charge
Good luck.
There is a reason many cheap chargers are timer based. Unlike, say, Lithium-Ion cells, cell voltage has little to say about charge states - 1.0V is usually described as the voltage of an 'empty' cell while the nominal voltage of a 'full' cell is typically 1.2V. (Incidentally, this is an advantage in some systems as a low voltage drop simplifies circuit design.)
According to Battery University good quality chargers use "a combination of voltage drop at full charge (negative delta V), rate-of-temperature-increase (dT/dt), absolute temperature and timeout timers. The charger utilizes whatever comes first to terminate the fast-charge."
Memory Effects
Hell if I know.
I've heard some people say NiCads need to be put through a full cycle once every month or so in order to fend off memory effects, and I've heard others claim that the whole thing is a myth. The truth seems to be somewhere in between, involving anecdotal evidence regarding orbiting satellites. Read more here.
Self-Discharge
The general consensus seems to be that the average cell loses about 10% of its charge per month.
Nickle-Metal Hydride (NiMH)
The NiMH chemistry is very similar to the NiCad chemistry - for example, they have the same nominal voltage (1.2V) and have similar charging requirements. NiMH cells are superior to to NiCads in exactly two ways: they have between two to four times the energy density (in terms of energy per unit mass), and they are either non-toxic or only mildly toxic (depending on who you talk to). That said, in every other metric NiCad cells are either on par with or are superior to NiMH cells. One example is cycle life: modern NiCads can be cycled thousands of times with very little drop in capacity, meanwhile NiMH have a life measured in the tens to hundreds of cycles.
Generally speaking, NiMH chemistry is a stop-gap solution while Lithium Ion technology is developed and implemented.
Quick and Dirty Charging & Terminating Charge
Due to similarities in chemistry, in many cases NiMH chargers can be used to charge NiCads (although your mileage may vary) although not the other way around.
While the difference between a discharged and charged NiCad cell is around 200mV, a NiMH has a difference of about 12mV. Add in fluctuations due to heating and cell aging, and it is practically impossible to reliably terminate charging at rates below 1C (the "Fast" charge method detailed above in the NiCad section). NiMH don't accept trickle charging well either; charge rate should not exceed 0.05C.
My recommendation would be to stick with a 1C charge rate at nominal cell voltage (1.2V per cell), and to only charge fully depleted cells.
Self-Discharge
The general consensus seems to be that NiMH have a discharge rate about 50% greater than NiCads; in otherwords, about 15% of its charge per month.
LiMnxNiyCozO2 / LiCoO2 (Lithium Ion, standard and polymer)
The Lithium Ion chemistry is the power source for virtually all modern portable electronics. Its high energy density, relatively simple charging requirements, ease of end-of-charge detection, respectable cycle life (modern cells should be above 80% capacity after about 300 cycles), lack of any memory effects, and very low self-discharge rate make it ideal for this purpose. The downside is the cells tend to be a bit unstable - they require specialized electronics to prevent damage or fire from over charging or over discharging, and are not physically robust.
A few things to note: So-called Lithium Polymer cells are simply a different way of packaging the cell, and technically should be called Lithium Ion Polymer cells. Paraphrasing Wikipedia: instead of holding the the lithium-salt electrolyte in an organic solvent, it is held in a solid polymer composite. Also, the LiCoO2 chemistry has a slightly higher power density than the LiMnxNiyCozO2 chemistry, and subsequently it is slightly more unstable.
Charging & Terminating Charge
Lithium Ion cells have a nominal voltage of 3.7V. A cell is considered empty when it reaches 2.4V (discharging to a higher voltage, say 2.5V, may increase the lifespan of the cell).
To charge a cell, apply a current less than the capacity (usually C/10 is recommended) at 4.20 volts. When the voltage of the cell reaches 4.20, disconnect it from the charger. That's it.
Applying a very low current to a severely depleted cell to bring its charge level up into nominal range before applying the normal charge current may extend the life of the cell.
Do not trickle charge Lithium Ion cells. Their extremely low self-discharge rate means they don't accept excess charge at all.
All Lithium Ion cells available to (and designed to be accessed by) the general consumer (i.e. camera, cell phone, and laptop batteries) have built in electronics that prevent over charging and over discharging of any internal cells. If the cell you are charging has built in protection electronics, what you will probably see is the voltage hit 4.20V and then very soon after the current through the cell will drop to almost nothing. This would be caused by the cell protection circuitry kicking in.
Any Lithium Ion pack with multiple cells in series will require some kind of charge balancer. A charge balancer ensures that all the cells in a series are at roughly the same charge level (which can be determined via output voltage). Consider: If we were to only use the primary power connections when charging a pack, to prevent damage to cells charging must stop as soon as any cell in the string reaches full charge, and discharging must stop when any cell is fully discharged. Thus, in order to maximize power output cells must be maintained as close to equal charge level as possible.
That said, maximum the capacity of a series is limited to the status of the cell in the worst condition: Say you had a series of a two cells, both rated at 500mAh, but one had been used elsewhere prior to being integrated into the pack and had degraded to 80% of its original capacity. The maximum amount of power one could take from the series before one of the cells was fully discharged would be 400mAh (80% of 500mAh). This would leave the other cell with about 100mAh remaining. This is why it is inadvisable to mix old cells with new cells and/or to mix cells of various capacities: the capacity of the series will be limited to the capacity of the cell with the lowest capacity.
(Don't ask me why a cell with a lower charge level than the other cells it is in series with doesn't get 'topped up' by the other cells while the pack is in use - I don't know.)
If the battery is a pack with multiple cells and internal electronics, the electronics will probably also act as a charge balancer in one of three ways:
It will cut off individual cells from the current source as they reach full charge
It will get one or more of the cells to full charge, disconnect from the charger, and then selectively dump current from one cell into another to bring all charge levels to about the same level
It will get one or more of the cells to full charge, disconnect from the charger, and then determine what the charge level is of the weakest cell is and drain the other cells down to that level
(Please note that the above three methods are educated guesses based on observations of various Lithium Ion battery packs and chargers.)
Any pack that requires some idle time between charging and use almost definitely uses one of the latter two methods.
Any Lithium Ion packs with multiple cells in parallel will either have specialized electronics to manage each cell or the cells in the pack are very carefully matched (at the very least they would need to have identical capacities - there may be other characteristics that also need to be matched).
Specialty retailers and R&D suppliers may offer Lithium Ion packs that have no internal electronics. These packs can be identified by an extra bundle of wires coming out of the pack, usually of smaller gauge than the primary power connections. These extra leads are connected to the junctions between cells in the pack (thus giving access to each cell in the pack) and are used by battery chargers/balancers to (surprise) charge and balance the battery pack.
Safety
Do not discharge cells below 2.4V - doing so may damage the cell. Do not charge the cell after it has reached 4.2V, or at a voltage higher than 4.2V - doing so may cause it to explode. Entertaining? Of course. Safe? Probably not.
Generally speaking cells should not become hot when charging or discharging.
DISCLAIMER
All content is owned by its respective creators/licence holders.
Unless otherwise stated, everything else: Copyright © 2010, Chlazza